A review by Wang Xianying and others from Shanghai Institute of Silicic Acid: Research progress of MOF-derived non-precious metal materials in zinc-air batteries
Metal-organic frameworks (MOFs) are porous crystalline materials with periodic structures formed by the coordination of metal ions or clusters with organic ligands. So far, MOFs materials have been widely used in the fields of catalysis, energy storage, conversion, gas adsorption and separation. Compared with MOFs, MOFs derivatives can not only effectively retain the advantages of MOFs porosity and high specific surface area, but also have better conductivity and stability, and can be applied to oxygen electrochemical reactions and related energy storage devices. Aiming at the oxygen-catalyzed reactions (oxygen reduction reaction and oxygen evolution reaction) involved in zinc-air batteries, this article reviews the research and application progress of non-noble metal materials derived from MOFs in zinc-air batteries.
Highlights of this article
1. A systematic review of the research and application progress of non-precious metal materials derived from different types of metal organic frameworks (MOFs) in zinc-air batteries.
2. Explained the insights into the material design strategy and its structure-performance relationship.
3. The challenges and future development prospects of MOFs-derived oxygen electrocatalysts in zinc-air batteries are proposed.
Introduction
Researcher Wang Xianying, Associate Researcher Yan Ya, Shanghai Institute of Ceramics, Chinese Academy of Sciences; Professor Xia Baoyu, Huazhong University of Science and Technology, etc.In this article, the basic principles of oxygen catalysis in zinc-air batteries are briefly introduced, and then non-metallic carbon materials, monoatomic materials, metal clusters/carbon composite materials and metal compounds/carbon composite materials are derived from MOFs. The research progress of non-noble metal oxygen electrocatalysts derived from MOFs in zinc-air batteries is systematically reviewed. By discussing the performance of these MOF-derived catalysts in oxygen reduction and oxygen evolution reactions and their service level in zinc-air batteries, we further reveal the design strategies and structure-performance of various MOF-derived non-noble metal oxygen electrocatalysts. The relationship between. Finally, the application prospects of MOF-derived oxygen electrocatalysts in zinc-air batteries are prospected.
Graphic guide
INon-metallic carbon materials derived from MOFs
Non-metallic carbon materials derived from MOFs have attracted widespread attention in the field of catalysis in recent years. Yang et al. grew ZIF-8 crystal particles in situ on the surface of silica microspheres and then carbonized them to remove the template, forming an ordered macroporous carbon framework (BHPC, Figure 1a) between the spherical voids. Using BHPC-950 as the air electrode, the assembled zinc-air battery can not only work at a high rate of 120 mA cm, but also provide an excellent capacity of 770 mAh g(Figure 1b). This indicates that the highly exposed graphite N derived from the template has unique texture characteristics and can promote the cathode oxygen reduction reaction of the zinc-air battery. Chai et al. used the composite of ZnO and ZIF-8 as a sacrificial template to prepare nitrogen-doped hollow carbon microspheres (Figure 1c). The large pore volume and high specific surface area of the microspheres not only promote the diffusion of electrolyte and gas molecules, but also provide abundant active centers. In addition, adding glucose as an additional carbon source in ZIF can increase the degree of graphitization of the sample and help remove zinc metal and zinc compound impurities, providing an effective way for the synthesis of MOF-derived metal-free nitrogen-doped porous carbon. Qian et al. reported that BN double-doped porous carbon (BNPC) thermally decomposes Zn-MOF (MC-BIF-1S) in a H₂-Ar mixed atmosphere for ORR/OER catalysis and decomposes into a BNPC solid with a porous structure (Figure 1d) ). The cycle stability of the assembled battery was tested for up to 100 hours without significant performance loss (Figure 1e).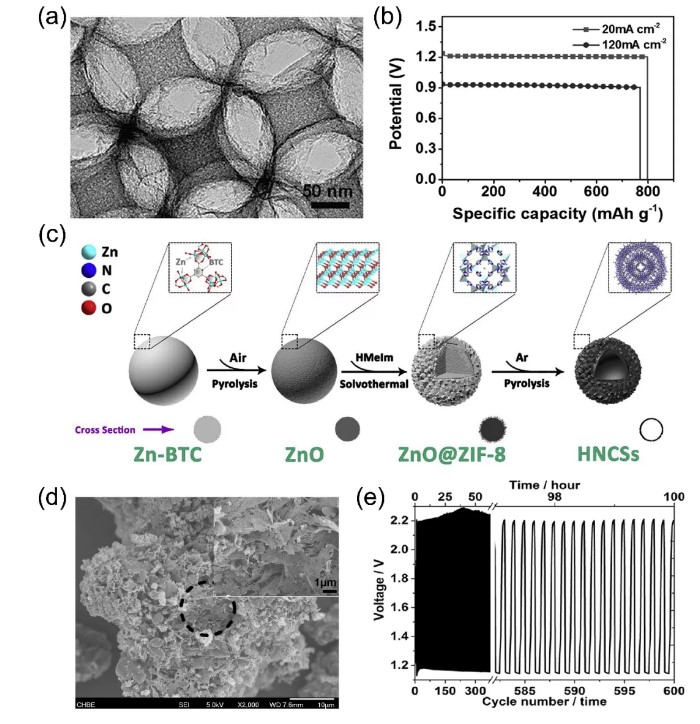
Figure 1. (a, b) TEM image of MOF-derived nitrogen-rich carbon crystal (BHPC-950) and specific capacitance of zinc-air battery; (c) Principle of preparing nitrogen-doped hollow carbon spheres by in-situ growth and calcination strategy Figure; (d, e) Scanning electron microscope image of BNPC-1100 and cycle performance graph of rechargeable zinc-air battery using BNPC-1100 as the air cathode.
II MOFs derived monoatomic catalyst materialsThe structural characteristics of MOFs themselves provide huge advantages for the synthesis of single-atom catalysts. Li et al. used the ZnS/ZIF-67 composite as a heat treatment template, and the carbonized ZnS self-sacrificed to form a nanorod structure, and at the same time provided a sulfur source for the formation of Co₉S₈ nanoparticles (Figure 2a). The obtained CoSA+Co₉S₈/HCNT has good oxygen electrocatalytic activity, and its potential difference (the difference between Ej=10 and E1/2) is 0.705 V, which is much smaller than the potential difference of Pt/C+RuO₂ (0.777 V). The peak power density of the liquid zinc-air battery assembled with CoSA+Co₉S₈/HCNT reaches 177.33 mW cm, which is much better than Pt/C+RuO₂ (Figure 2b). At 1 mA cm, the flexible zinc-air battery based on CoSA+Co₉S₈/HCNT catalyst still maintains a stable charge/discharge potential after a long cycle, and has excellent stability (Figure 2c). Wang et al. reported a hollow structure composed of a single iron atom on a N, P, and S co-doped hollow carbon polyhedron (Fe-SAS/NPS-HC) constructed from a MOF@polymer composite material. They emphasized that the polymer-based coating modulates the electrons in the active metal center through the Kirkendall effect, while the coordination of N and the remote modulation of S and P promote the construction of the hollow structure (Figure 2d). In order to further enhance the oxygen electrochemical reaction of the Fe-N active center in the zinc-air battery, Suns team used ZIF-8 to combine Fe2+ and Fe-Phen into a nanocage in situ during its growth process to synthesize monoatomic Fe. -NX-C electrocatalyst (Figure 2e). Since the Fe-Phen species provides both Fe2+ and organic ligands (Phen), the Fe-Phen@ZIF-8 precursor plays an important role in the preparation of single-atom catalysts. In addition, Hou et al. modified single-atom iron sites for ORR catalysis through a silica-mediated MOF template method (Figure 2f). The prepared oxygen catalyst showed good performance in zinc-air batteries with maximum capacity It is 807.5 mAh g-1, the peak power density is 186.8 mW cm-2, and the energy density reaches 962.7 Wh kg-1, which is equivalent to the performance of the precious metal Pt/C catalyst (Figure 2g, h).
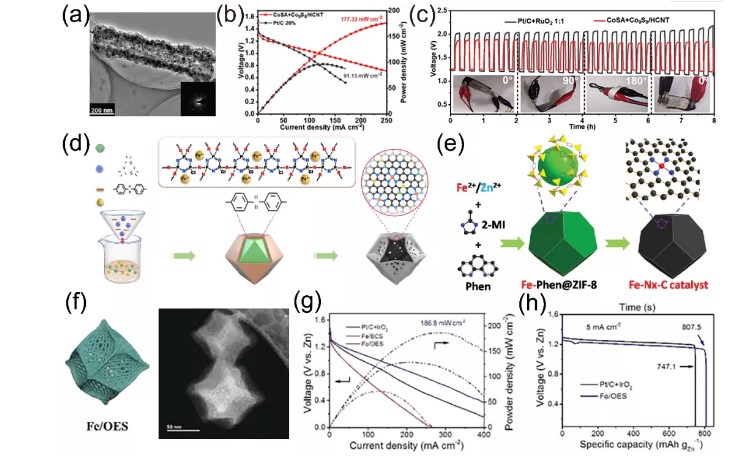
Figure 1. (a, b) TEM image of MOF-derived nitrogen-rich carbon crystal (BHPC-950) and specific capacitance of zinc-air battery (c) Principle of preparing nitrogen-doped hollow carbon spheres by in-situ growth and calcination strategy Figure;(d, e) Scanning electron microscope image of BNPC-1100 and cycle performance graph of rechargeable zinc-air battery using BNPC-1100 as the air cathode.
IIMOFs derived monoatomic catalyst materialsThe structural characteristics of MOFs themselves provide huge advantages for the synthesis of single-atom catalysts. Li et al. used the ZnS/ZIF-67 composite as a heat treatment template, and the carbonized ZnS self-sacrificed to form a nanorod structure, and at the same time provided a sulfur source for the formation of Co₉S₈ nanoparticles (Figure 2a). The obtained CoSA+Co₉S₈/HCNT has good oxygen electrocatalytic activity, and its potential difference (the difference between Ej=10 and E1/2) is 0.705 V, which is much smaller than the potential difference of Pt/C+RuO₂ (0.777 V). The peak power density of the liquid zinc-air battery assembled with CoSA+Co₉S₈/HCNT reaches 177.33 mW cm-2, which is much better than Pt/C+RuO₂ (Figure 2b). At 1 mA cm-2, the flexible zinc-air battery based on CoSA+Co₉S₈/HCNT catalyst still maintains a stable charge/discharge potential after a long cycle, and has excellent stability (Figure 2c). Wang et al. reported a hollow structure composed of a single iron atom on a N, P, and S co-doped hollow carbon polyhedron (Fe-SAS/NPS-HC) constructed from a MOF@polymer composite material. They emphasized that the polymer-based coating modulates the electrons in the active metal center through the Kirkendall effect, while the coordination of N and the remote modulation of S and P promote the construction of the hollow structure (Figure 2d). In order to further enhance the oxygen electrochemical reaction of the Fe-N active center in the zinc-air battery, Suns team used ZIF-8 to combine Fe and Fe-Phen into a nanocage in situ during its growth process to synthesize monoatomic Fe. -NX-C electrocatalyst (Figure 2e). Since the Fe-Phen species provides both Fe and organic ligands (Phen), the Fe-Phen@ZIF-8 precursor plays an important role in the preparation of single-atom catalysts. In addition, Hou et al. modified single-atom iron sites for ORR catalysis through a silica-mediated MOF template method (Figure 2f). The prepared oxygen catalyst showed good performance in zinc-air batteries with maximum capacity It is 807.5 mAh g-1, the peak power density is 186.8 mW cm-2, and the energy density reaches 962.7 Wh kg-1, which is equivalent to the performance of the precious metal Pt/C catalyst (Figure 2g, h).
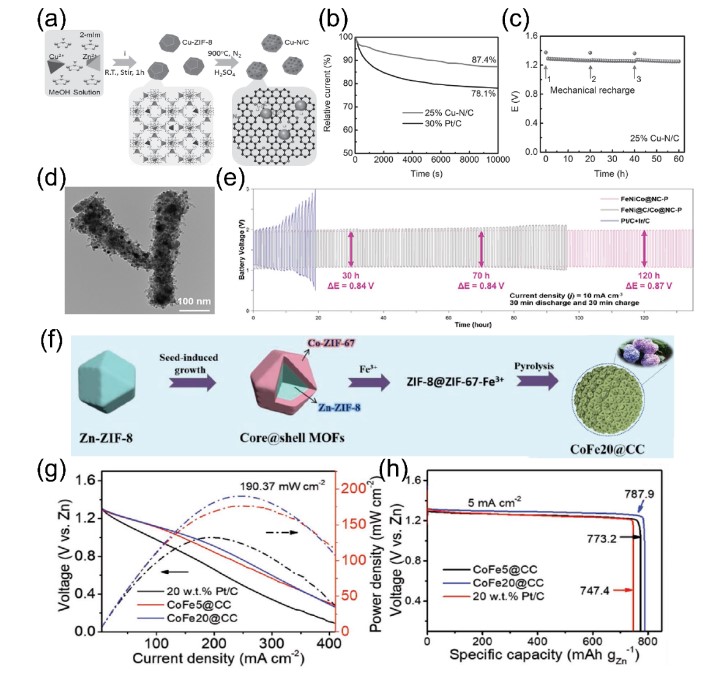
Figure 3. (ac) Cu-N/C catalyst synthesis process, ORR current-time response and zinc-air battery performance; (d) TEM image of FeNiCo@NC-P catalyst; (e) FeNiCo@NC-P application In the cycle stability of zinc-air battery; (fh) the synthetic schematic diagram of the open carbon cage forming the embroidered spherical structure, the power density map and the specific capacity of the zinc-air battery.
IVMOF derived metal compound/carbon composite material
In order to further design oxygen electrocatalysts for zinc-air batteries with high-efficiency service level and lifetime, using MOFs as platform materials, designing metal compounds/carbon composites has become a research hotspot in recent years. For example, Guan et al. designed a nitrogen-doped carbon nanoarray (NC-Co₃O₄) embedded with irregular hollow Co₃O₄ nanospheres using a MOFs precursor with a special structure. During the carbonization process, the surface of the metal nanoparticles is covered with a layer of graphitic carbon (Figure 4a), which suppresses the Kirkendall effect on the nanoscale, promotes the formation of irregular hollow Co₃O₄ nanospheres, and has good catalytic performance for OER and ORR . In addition, the integrated NC-Co₃O₄/CC can be directly used as the additive-free air cathode of a flexible all-solid-state zinc-air battery, showing a large capacity of 387.2 mAh g-1 (Figure 4b). In order to further study the application of this composite material in oxygen electrolysis, Zhao et al. constructed a new type of Co-MOF by directly carbonizing SPDP and H2BDC ligand (1,4-phthalic acid), and further prepared N with embedded Co₉S₈ nanoparticles. , O and S doped carbon matrix (Co₉S₈@TDC, Figure 4c). The intrinsic activity of Co₉S₈ nanoparticles and the heteroatom-doped carbon shell promote the catalytic performance of OER and ORR. Co₉S₈@TDC-900 is used as the air cathode catalyst layer of rechargeable zinc-air batteries, providing a fairly high open circuit voltage (1.5 V) and long-term charge and discharge stability (Figure 4d). Considering the excellent flexibility, ultra-high specific surface area, layered pore structure and high catalytic activity of MOF and metal hydrogen disulfide, Mus team reported an efficient electrocatalyst based on vertically arranged MoS₂ nanosheets. A layered Mo-N/C framework is formed by carbonizing ZIF-8 to form an interface Mo-N coupling center (Figure 4e). When it is used as the cathode electrocatalyst of a zinc-air battery, its power density is approximately 196.4 mW cm-2 (Figure 4f). This good electrocatalytic performance is attributed to the synergy of unique chemical composition, three-phase active center, and layered pore structure that is conducive to rapid mass transfer. Wei et al. used MOF envelope protein and melamine as raw materials to fix iron-nickel phosphide nanoparticles on the envelope by pyrolysis, and rely on carbon nanotubes to connect to the carbon to prepare an N-doped envelope carbon-based framework (Figure 4g). The synergistic effect between the carbon skeleton and the highly exposed phosphide sites makes the material exhibit highly efficient and multifunctional electrocatalytic performance in HER, OER and ORR, and is also a qualified zinc-air battery catalyst.
Figure 4. (a, b) Preparation process and voltage-capacity curve of vertical NC-Co₃O₄ array on flexible carbon cloth (solid zinc-air battery); (c, d) SEM images and long-term images of Co₉S₈@TDC-900 catalyst Charge-discharge cycle diagram; (e, f) Mo-N/C@MoS₂ catalyst synthesis schematic and VI polarization and power density curve; (g) FeNiP/NCH catalyst preparation process flow chart.
V Summary and outlook
In summary, although MOF-derived non-noble metal oxygen electrocatalysts have made great progress in the field of zinc-air batteries, there are still some urgent problems to be solved. First of all, the design and preparation of high-efficiency electrode materials has always been the top priority. The structural diversity of MOF makes the material system very complicated. Choosing a suitable MOF precursor is the key to designing MOF-derived oxygen electrocatalytic materials. Using high-throughput technology, people can calculate and predict the effects of different MOF components and structures on the performance of the final product, thereby accelerating the research progress of MOF-derived air electrode materials. Secondly, accurate structural analysis of the prepared MOFs derivatives is particularly important for studying their structure-activity relationships in the oxygen electrocatalysis process of zinc-air batteries. Through the combination of electrode and battery testing and advanced in-situ characterization technology, the structural evolution of the catalyst during the electrochemical reaction and battery use can be fully characterized. Finally, the commercialization of zinc-air batteries needs to be further balanced in terms of performance, safety and cost. By optimizing the electrode material, battery device, electrolyte and assembly process, the power density, energy density and cycle performance of the battery can be improved. Achieving a balance between cost and performance is the main way to promote zinc-air batteries to commercial applications.
Written by: original author
Editor: Nawei Express (English)Editorial Department
This information is sourced from the Internet for academic exchanges. If there is any infringement, please contact us to delete it immediately
18915694570
Previous: NML Review | Professor