Professor Sun Qi of Zhejiang University Nature: Artificial thermal skin is here! Research on COF Nanofluidic Film as a Highly Sensitive Bionic Thermal Sensing Platform
Sensing temperature is essential for organisms to maintain normal life activities. In mammals, thermal stimuli are converted into electrochemical potentials through thermo-TRP ion channels, and then converted into action potentials through nerve cells, such as the generation of pain. From the perspective of bionics, the conduction of thermal signals is simulated in vitro, and appropriate nanochannels are designed to convert chemical signals into electrical signals, or thermally active molecules are implanted in artificial systems to adjust thermal sensation, and a bionic "thermometer" is designed. The research hotspot of scientists for a long time. In addition, the realization of reversible thermal responsiveness and only works when the temperature reaches the thermal transition temperature, limiting the temperature response to a small range has become a research difficulty.
The team of Professor Sun Qi of Zhejiang University and the team of Professor Shengqian Ma of the University of North Texas have creatively prepared a nanofluidic membrane based on an ionic covalent organic framework (COF), which can intelligently monitor temperature changes and The form of continuous potential difference is displayed. The sub-nano channel has a high density of charge sites, which makes the membrane layer have high selectivity and thermal sensitivity of up to 1.27 mV K-1. The system also has the advantages of good salt tolerance, wide operating temperature, synchronous response to temperature stimuli, and long-term super stability. The research was published in the latest issue of "Nature Communications" as a paper entitled "Covalent organic framework nanofluidic membrane as a platform for highly sensitive bionic thermosensation".
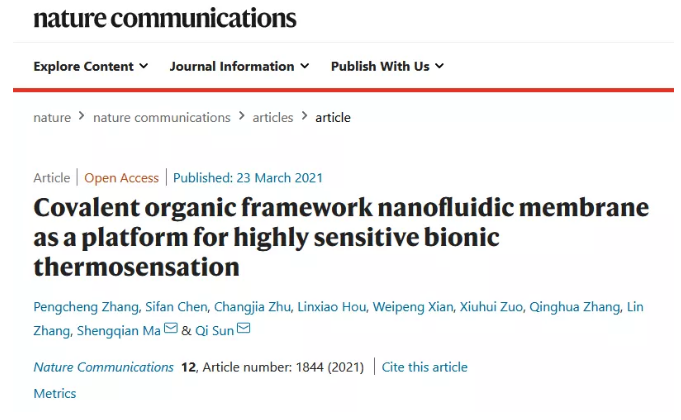
[Construction of COF fluid control membrane]
The nanofluid mass transfer formula helps us better understand and simulate the function of biofilm pores. According to this equation, the thermoelectric response to temperature changes can be expressed as a continuous change in electric potential, which is consistent with what is observed in nature. It can be inferred that under the same other conditions, the cation selectivity of the nanofluidic device is a key parameter to produce a significant potential difference response and temperature change. The membrane pore size is one of the key factors that determine the selectivity of the membrane; only when the inner diameter is smaller than the thickness of the electric double layer of the solution, it can play an effective ion shielding effect. Therefore, in order to improve the thermal sensation performance, it is very important to develop a membrane pore structure that can simultaneously control the pore size and fixed charge position. Two-dimensional COFs have become the best candidates with their designable channels. In addition, the two-dimensional flakes can be superimposed to produce a three-dimensional film under the drive of three-dimensional π-stacking, thereby providing a direct transmission channel with nanopores. COFs are ideal materials for constructing nanofluidic membrane devices.
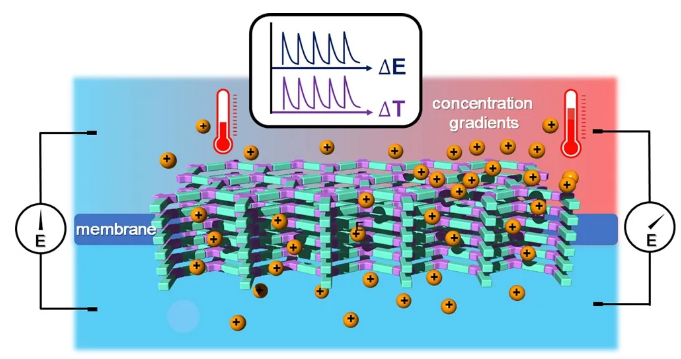
Figure 1 Ionic covalent organic framework nanofluidic film as a biomimetic thermometer
High ion density is conducive to generating a unipolar ion environment, and the Debye shielding length is a characteristic length that depends on the ionic strength of the solution (the higher the ionic strength, the smaller the Debye length). Therefore, the smaller the COF pore size, the higher the charge density, and the better the selectivity. In order to imitate the thermo-TRP ion channel, COF synthesized by 1,3,5-triformylphloroglucinol (Tp) and triaminoguanidine hydrochloride (Tag) was selected as the nanofluidic system (Fig. 2a, b).
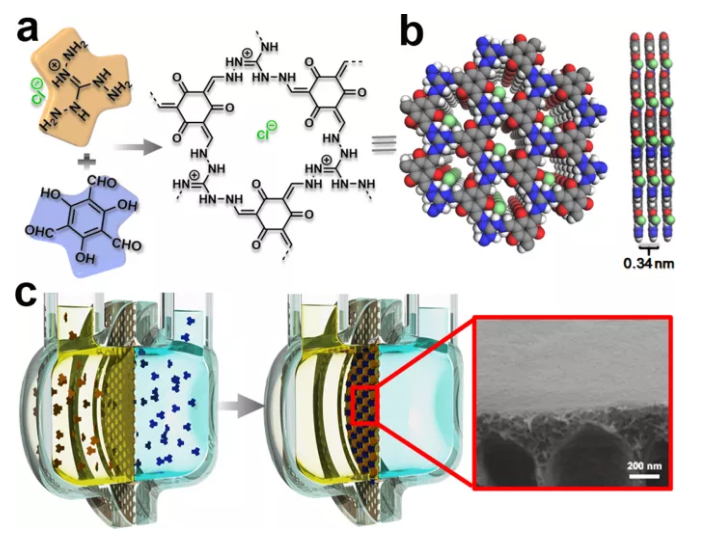
Figure 2 (a) Synthesis of Tp Tag-COF by condensation of Tp and Tag. (b) Tp Tag-COF stacking results (blue, N; gray, C; red; O; white, H; green, Cl). c Tp Tag-COF/PAN schematic diagram and SEM image.
【Preparation and Characterization of Membrane】
The author prepared the COF base film by acid-catalyzed interfacial polymerization. PAN acts as a support layer because it is hydrophilic and negatively charged, which can reduce the transmembrane energy of cations. A yellow film is formed on the organic phase side of the PAN surface, which is a COF nano film. The scanning electron microscope image shows a smooth, crack-free, continuous film surface with a thickness of ~100 nm. The attenuated total reflection infrared (ATR-IR) analysis confirmed the successful formation of the β-ketoenamine structure of the COF film. Under the environment of 1 mM KCl (pH ~7), the zeta potentials of Tp Tag-COF/PAN membrane and PAN membrane are 40.88 mV and 51.1 mV, respectively. X-ray diffraction of the powder of the self-supporting Tp Tag-COF film showed that there are two relatively broad peaks at 2θ values of ~11° and ~28°, corresponding to (100) and (001) crystal planes, respectively. In order to clarify the composition of the COF framework, a theoretical simulation was carried out using Materials Studio, and the c-axis aperture was 0.8 nm.
In order to determine the selectivity of Tp Tag-COF/PAN, the reversal potential under different KCl concentration ranges was evaluated. KCl was chosen because the mobility of K+ and Cl- are very similar. When cis/trans = 1 mM/0.1 mM, 10 mM/1 mM and 100 mM/10 mM KCl aqueous solutions, the X-axis intercept (Vr) of the current-voltage curve gives reversal potentials of 57.9, 55.8, and 43.4 mV (Figure 3a, cis refers to the surface of the active COF membrane). Under the concentration gradient of 100 mM/10 mM NaCl, LiCl, and MgSO4, the transmission ratios (selectivity) of Na+/Cl-, Li+/Cl-, Mg2+/SO42- are calculated to be 10, 8, and 3.5, respectively. The difference in selectivity can be explained by different ion diffusion rates. In order to further understand the transport behavior of ions, the author performed molecular dynamics (MD) simulations. The Tp Tag-COF layer is sandwiched between KCl aqueous solution and deionized water. As shown in Figure 3, it shows that only water molecules and K+ can move, thus confirming that the K+ transmittance is higher than that of Cl-.
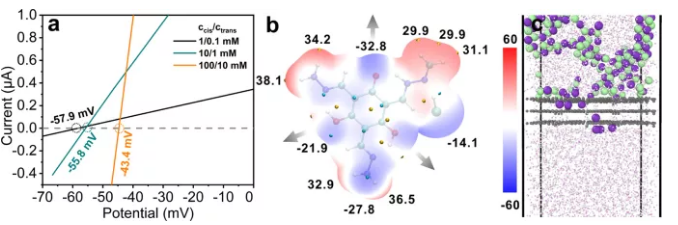
Figure 3 (a) Tp Tag-COF/PAN (black, 1/0.1 mM; green, 10/1 mM; orange, 100/10 mM KCl solution). (B) The surface electrostatic potential (ESP) of van der Waals and the area percentage within each ESP range. The local minimum and maximum values of the effective surface of the ESP are represented by red and blue spheres and marked with numbers. (C) The ion transport operation of the TpTag-COF sub-nano channel is a schematic diagram. MD simulation shows that the K+ transmittance of Tp Tag-COF/PAN film is higher than Cl- (purple, K; green, Cl; red, O; white, H; gray, Tg Tag-COF layer).
[Characterization of the thermoelectric response of the film]
The author further studied the thermoelectric responsiveness of Tp Tag-COF/PAN. The membrane is placed between the two temperature control chambers of the permeation chamber. The temperature gradient is controlled at ~10 K. Thermal stimuli drive the movement of ions, creating a potential difference at the boundary of the membrane and recording. A thermocouple is used to monitor the difference between the two room temperatures in real time, and the Ag/AgCl electrode detects the open circuit voltage (Voc). The ion selective permeability depends on the solution concentration; therefore, the effect of KCl concentration on the thermoelectric response performance of Tp Tag-COF/PAN was first evaluated. In order to study the sensitivity of thermal sensation, two chambers were filled with KCl aqueous solution in the range of 0.5-100 mM, and a similar trend over time was observed (Figure 4a). In order to highlight the role of the continuous regular pores of COF, the author compared the thermal sensitivity of Bt Tag/PAN film synthesized by condensation of 1,3,5-benzenetricarbonyl (Bt) on PAN and Tp Tag-COF/PAN ( Supplementary materials).
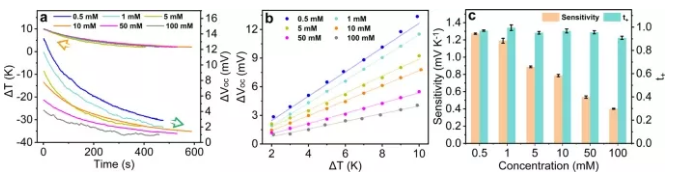
Figure 4 Thermal response performance of Tp Tag-COF/PAN in different concentrations of KCl aqueous solution (0.5-100 mM).
[Preparation of wearable temperature sensor]
Based on these results, the author explored the potential of Tp Tag-COF/PAN in the design of wearable devices with thermal sensory capabilities. As a sensor, the membrane is placed between carbon cloth immersed in a KCl solution (1 mM). The temperature gradient is induced by simply heating or cooling a piece of carbon cloth, and the measurement is performed using the method described above. The resulting potential change is detected by the Ag/AgCl electrode. When the system returns to thermal equilibrium, another temperature gradient is applied to detect dynamic potential changes. Figure 5 shows the real-time output potential response of the thermal sensor system, demonstrating its sensitivity and repeatability. In addition, the output potential changes in response to different temperature gradients also have significant differences. In general, the developed thermal sensor system shows great application potential in the design of wearable temperature sensors.
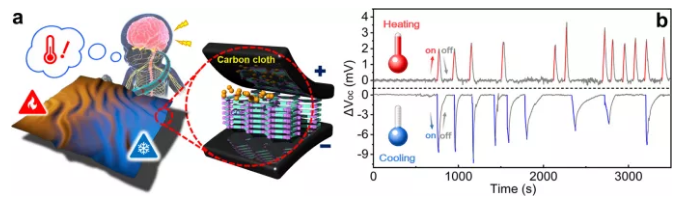
Figure 5 (a) Schematic diagram of designing smart textiles with thermal sensation ability. (b) Real-time measurement of potential changes, corresponding to the applied temperature gradient (red, heating; gray, heating/cooling; blue, cooling).
In short, the authors prepared COFs membranes as high-efficiency thermal sensors, and used the ion selective permeability of the membranes to prove that COF sub-nanochannels can simulate thermally responsive ion channels observed in nature. The author stated that based on the results of this study, an artificial skin that can detect ambient temperature is currently being developed. This work has taken an important step in the development of thermosensitive devices.
The team of Professor Sun Qi of Zhejiang University and the team of Professor Shengqian Ma of the University of North Texas have creatively prepared a nanofluidic membrane based on an ionic covalent organic framework (COF), which can intelligently monitor temperature changes and The form of continuous potential difference is displayed. The sub-nano channel has a high density of charge sites, which makes the membrane layer have high selectivity and thermal sensitivity of up to 1.27 mV K-1. The system also has the advantages of good salt tolerance, wide operating temperature, synchronous response to temperature stimuli, and long-term super stability. The research was published in the latest issue of "Nature Communications" as a paper entitled "Covalent organic framework nanofluidic membrane as a platform for highly sensitive bionic thermosensation".
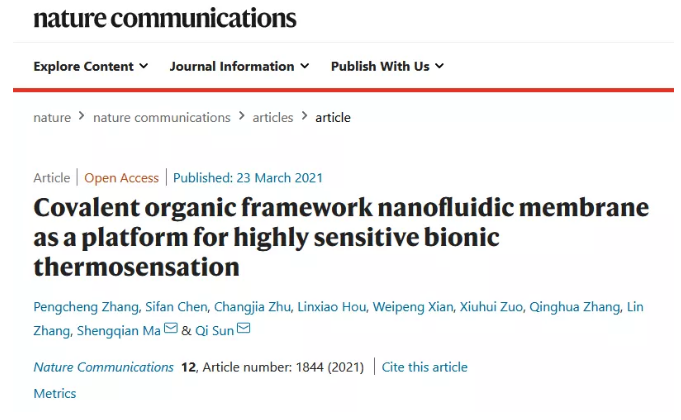
[Construction of COF fluid control membrane]
The nanofluid mass transfer formula helps us better understand and simulate the function of biofilm pores. According to this equation, the thermoelectric response to temperature changes can be expressed as a continuous change in electric potential, which is consistent with what is observed in nature. It can be inferred that under the same other conditions, the cation selectivity of the nanofluidic device is a key parameter to produce a significant potential difference response and temperature change. The membrane pore size is one of the key factors that determine the selectivity of the membrane; only when the inner diameter is smaller than the thickness of the electric double layer of the solution, it can play an effective ion shielding effect. Therefore, in order to improve the thermal sensation performance, it is very important to develop a membrane pore structure that can simultaneously control the pore size and fixed charge position. Two-dimensional COFs have become the best candidates with their designable channels. In addition, the two-dimensional flakes can be superimposed to produce a three-dimensional film under the drive of three-dimensional π-stacking, thereby providing a direct transmission channel with nanopores. COFs are ideal materials for constructing nanofluidic membrane devices.
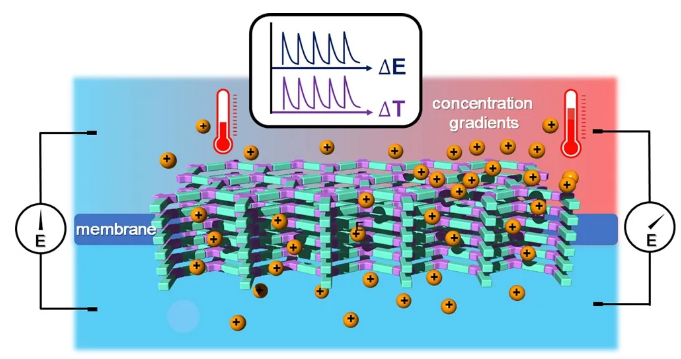
Figure 1 Ionic covalent organic framework nanofluidic film as a biomimetic thermometer
High ion density is conducive to generating a unipolar ion environment, and the Debye shielding length is a characteristic length that depends on the ionic strength of the solution (the higher the ionic strength, the smaller the Debye length). Therefore, the smaller the COF pore size, the higher the charge density, and the better the selectivity. In order to imitate the thermo-TRP ion channel, COF synthesized by 1,3,5-triformylphloroglucinol (Tp) and triaminoguanidine hydrochloride (Tag) was selected as the nanofluidic system (Fig. 2a, b).
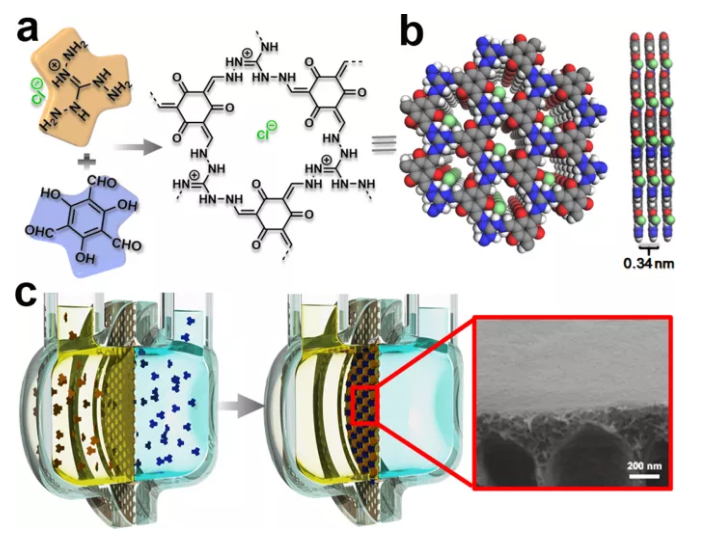
Figure 2 (a) Synthesis of Tp Tag-COF by condensation of Tp and Tag. (b) Tp Tag-COF stacking results (blue, N; gray, C; red; O; white, H; green, Cl). c Tp Tag-COF/PAN schematic diagram and SEM image.
【Preparation and Characterization of Membrane】
The author prepared the COF base film by acid-catalyzed interfacial polymerization. PAN acts as a support layer because it is hydrophilic and negatively charged, which can reduce the transmembrane energy of cations. A yellow film is formed on the organic phase side of the PAN surface, which is a COF nano film. The scanning electron microscope image shows a smooth, crack-free, continuous film surface with a thickness of ~100 nm. The attenuated total reflection infrared (ATR-IR) analysis confirmed the successful formation of the β-ketoenamine structure of the COF film. Under the environment of 1 mM KCl (pH ~7), the zeta potentials of Tp Tag-COF/PAN membrane and PAN membrane are 40.88 mV and 51.1 mV, respectively. X-ray diffraction of the powder of the self-supporting Tp Tag-COF film showed that there are two relatively broad peaks at 2θ values of ~11° and ~28°, corresponding to (100) and (001) crystal planes, respectively. In order to clarify the composition of the COF framework, a theoretical simulation was carried out using Materials Studio, and the c-axis aperture was 0.8 nm.
In order to determine the selectivity of Tp Tag-COF/PAN, the reversal potential under different KCl concentration ranges was evaluated. KCl was chosen because the mobility of K+ and Cl- are very similar. When cis/trans = 1 mM/0.1 mM, 10 mM/1 mM and 100 mM/10 mM KCl aqueous solutions, the X-axis intercept (Vr) of the current-voltage curve gives reversal potentials of 57.9, 55.8, and 43.4 mV (Figure 3a, cis refers to the surface of the active COF membrane). Under the concentration gradient of 100 mM/10 mM NaCl, LiCl, and MgSO4, the transmission ratios (selectivity) of Na+/Cl-, Li+/Cl-, Mg2+/SO42- are calculated to be 10, 8, and 3.5, respectively. The difference in selectivity can be explained by different ion diffusion rates. In order to further understand the transport behavior of ions, the author performed molecular dynamics (MD) simulations. The Tp Tag-COF layer is sandwiched between KCl aqueous solution and deionized water. As shown in Figure 3, it shows that only water molecules and K+ can move, thus confirming that the K+ transmittance is higher than that of Cl-.
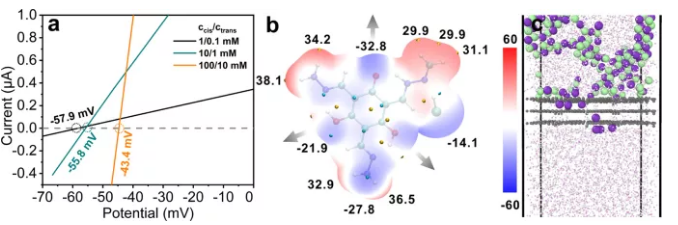
Figure 3 (a) Tp Tag-COF/PAN (black, 1/0.1 mM; green, 10/1 mM; orange, 100/10 mM KCl solution). (B) The surface electrostatic potential (ESP) of van der Waals and the area percentage within each ESP range. The local minimum and maximum values of the effective surface of the ESP are represented by red and blue spheres and marked with numbers. (C) The ion transport operation of the TpTag-COF sub-nano channel is a schematic diagram. MD simulation shows that the K+ transmittance of Tp Tag-COF/PAN film is higher than Cl- (purple, K; green, Cl; red, O; white, H; gray, Tg Tag-COF layer).
[Characterization of the thermoelectric response of the film]
The author further studied the thermoelectric responsiveness of Tp Tag-COF/PAN. The membrane is placed between the two temperature control chambers of the permeation chamber. The temperature gradient is controlled at ~10 K. Thermal stimuli drive the movement of ions, creating a potential difference at the boundary of the membrane and recording. A thermocouple is used to monitor the difference between the two room temperatures in real time, and the Ag/AgCl electrode detects the open circuit voltage (Voc). The ion selective permeability depends on the solution concentration; therefore, the effect of KCl concentration on the thermoelectric response performance of Tp Tag-COF/PAN was first evaluated. In order to study the sensitivity of thermal sensation, two chambers were filled with KCl aqueous solution in the range of 0.5-100 mM, and a similar trend over time was observed (Figure 4a). In order to highlight the role of the continuous regular pores of COF, the author compared the thermal sensitivity of Bt Tag/PAN film synthesized by condensation of 1,3,5-benzenetricarbonyl (Bt) on PAN and Tp Tag-COF/PAN ( Supplementary materials).
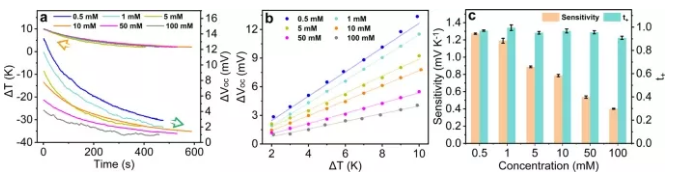
Figure 4 Thermal response performance of Tp Tag-COF/PAN in different concentrations of KCl aqueous solution (0.5-100 mM).
[Preparation of wearable temperature sensor]
Based on these results, the author explored the potential of Tp Tag-COF/PAN in the design of wearable devices with thermal sensory capabilities. As a sensor, the membrane is placed between carbon cloth immersed in a KCl solution (1 mM). The temperature gradient is induced by simply heating or cooling a piece of carbon cloth, and the measurement is performed using the method described above. The resulting potential change is detected by the Ag/AgCl electrode. When the system returns to thermal equilibrium, another temperature gradient is applied to detect dynamic potential changes. Figure 5 shows the real-time output potential response of the thermal sensor system, demonstrating its sensitivity and repeatability. In addition, the output potential changes in response to different temperature gradients also have significant differences. In general, the developed thermal sensor system shows great application potential in the design of wearable temperature sensors.
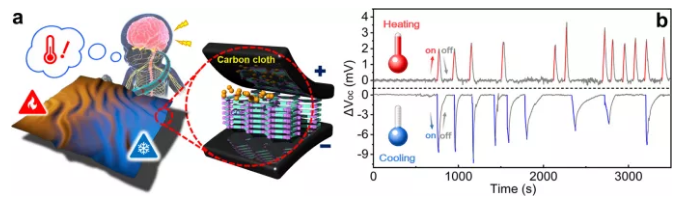
Figure 5 (a) Schematic diagram of designing smart textiles with thermal sensation ability. (b) Real-time measurement of potential changes, corresponding to the applied temperature gradient (red, heating; gray, heating/cooling; blue, cooling).
In short, the authors prepared COFs membranes as high-efficiency thermal sensors, and used the ion selective permeability of the membranes to prove that COF sub-nanochannels can simulate thermally responsive ion channels observed in nature. The author stated that based on the results of this study, an artificial skin that can detect ambient temperature is currently being developed. This work has taken an important step in the development of thermosensitive devices.
18915694570
Previous: ACS Nano from Beihua W