MIT Zhao Xuanhe "AM": Minimally invasive tissue-sealed multifunctional origami patch antibacterial hydrogel
For decades, because bioadhesive materials have the potential to replace sutures and staples to seal tissue during minimally invasive surgical procedures, they have attracted much attention. However, the complexity of delivering bioadhesives through narrow spaces and achieving strong bonds in a fluid-rich physiological environment continues to impose great limitations on the surgical translation of existing sealants. Recently, the team of Professor Hyunwoo Yuk/Zhao Xuanhe of the Massachusetts Institute of Technology introduced a new strategy for minimally invasive tissue sealing based on a multilayer bioadhesive patch, which is designed to repel body fluids and form rapid pressure-triggered adhesion with wet tissue Strength, and resist biological fouling and inflammation. The related paper A Multifunctional Origami Patch for Minimally Invasive Tissue Sealing was published on "Advanced Materials". The multifunctional patch is realized by the synergistic combination of three different functional layers: i) micro-textured bioadhesive layer, ii) dynamic hydrophobic and hydrophobic fluid layer, and iii) antifouling zwitterionic non-adhesive layer. The patch can form firm adhesion to the tissue surface in the presence of blood, and exhibits excellent resistance to bacterial adhesion, fibrinogen adsorption and fibrin formation in the body. By adopting an origami-based manufacturing strategy, it is proved that the patch can be easily integrated with various minimally invasive end effectors to provide convenient tissue sealing in an isolated pig model, which is useful for performing microscopic operations in various clinical situations. Creating tissue seals provides new opportunities.
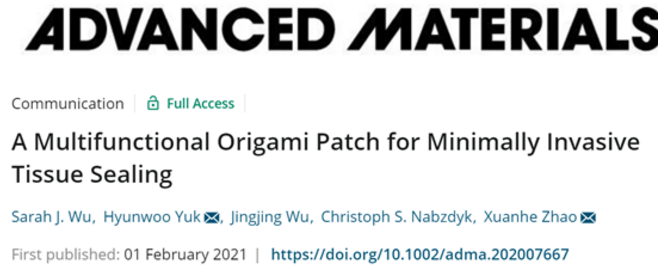
The author introduced a new tissue sealing and repair strategy based on the multilayer tissue sealing patch, which synergistically combines three core functions to solve the above limitations (Figure 1): body fluid resistance, strong pressure on wet tissue Need adhesion and anti-fouling behavior. In order to achieve these characteristics, the patch integrates three different functional layers: i) a micro-textured bioadhesive layer, ii) a dynamic, blood-repellent hydrophobic fluid layer, and iii) an antifouling zwitterionic non-sticky layer Attached. It is worth noting that the material properties of the multilayer patch make it suitable for origami-inspired manufacturing methods, thereby making it highly customizable. This ability to use custom form factors can be used for surgical applications through multiple deployment mechanisms driven by different surgical end effectors, providing promising solutions for various clinical indications (Figure 1b).
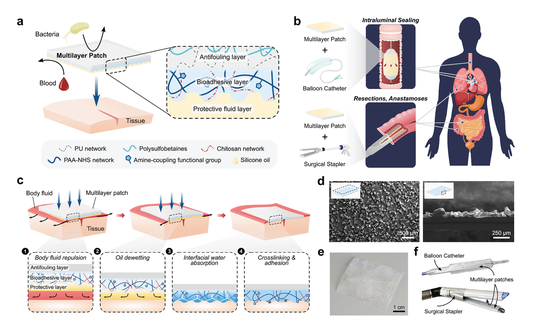
Figure 1 a) Schematic diagram of the multilayer composition of a bioadhesive patch. The patch includes a textured bioadhesive with an antifouling polymer layer fused on the non-adhesive side and wetted by a hydrophobic fluid layer on the adhesive side to repel body fluids. b) An exemplary minimally invasive surgery example of a multi-layer patch for intraluminal sealing of tubular organs and structures through a balloon catheter, and a surgical stapler for linear sealing in resection and anastomosis. c) Schematic diagram of the adhesion mechanism of the multilayer patch. (1) When the patch is moved toward the tissue, the hydrophobic protective layer repels blood and prevents contamination of the bioadhesive layer. (2) Applying a pressure exceeding 77.5 kPa will cause the oil to dewet from the bioadhesive layer. (3) The bioadhesive layer contacts the tissue surface and immediately absorbs the interface water, forming temporary crosslinks. (4) Covalent bonds are formed between the NHS ester functional groups in the bioadhesive layer to achieve stable long-term adhesion. d) Scanning electron microscope images of the top view (left) and side view (right) of the micro-textured surface of the bioadhesive layer. e) The photo of the assembled multilayer patch. f) Multi-layer patch loaded on balloon catheter and surgical stapler.
In order to evaluate the protective ability of the hydrophobic liquid layer, the authors exposed patch samples with or without silicone oil to blood and compared their fouling behavior (Figure 2a). When immersed in a pig blood bath, the patch without a silicone oil layer will be immediately wetted by blood and lose its adhesion ability, while the patch with a protective silicone oil layer will resist blood contamination and remain intact (Figure 2a). In order to further study the effect of surface microtexture on the stability of the fluid layer, the author vigorously shakes the multilayer membrane with a flat and microtextured bioadhesive surface in a pig blood bath. Although the multilayer patch with a flat bioadhesive surface showed a lot of blood contamination after shaking, the patch containing a micro-textured surface showed a strong protective effect on the bioadhesive layer to prevent strong blood flow ( Figure 2b), which supports the importance of the microtexture design of the bioadhesive layer. In order to obtain stable antifouling performance. The authors also measured the adhesion of pig skin tissues using various commercially available tissue adhesives (including fibrin-based Tisseel, albumin-based Bioglue, polyethylene glycol (PEG)-based Coseal and cyanoacrylate). Interface toughness, shear strength and tensile strength. Base tissue acrylic (Figure 2c). Compared with these commercially available tissue adhesives, the multilayer patch can resist blood contamination and significantly improve the interface toughness (536.7±93.4 J m-2), shear strength (56.1±4.7 kPa) and Tensile strength (65.0±8.0 kPa) (Figure 2c).
In order to reduce biofouling and postoperative inflammation, the authors integrated a zwitterionic interpenetrating elastomer layer on the non-adhesive surface of the patch (Figure 1a). It has been found that zwitterionic polymers have excellent antifouling properties. Their unique anti-fouling adsorption ability is attributed to the presence of cationic and anionic groups in the net neutral polymer chain. They promote the formation of a tightly hydrated shell while minimizing the hydrogen bond structure of free water molecules (Figure 3a) . The high energy consumption of this hydrated shell prevents the surface adsorption of bacteria and biomolecules, which are involved in inflammatory reactions such as infection, blood coagulation and postoperative adhesion formation. However, zwitterionic hydrogels generally have poor mechanical properties, such as low toughness and stretchability, which may be detrimental to their long-term robustness and stability in a dynamic physiological environment.
In order to characterize the antifouling performance of the zwitterionic layer, the authors studied the ability of the patch to reduce bacterial adhesion in vitro, fibrinogen adsorption in vitro and fibrin formation in vivo (Figure 3d-j). Bacteria adhering to the implant material can cause biofilm formation and infection of the surgical site. Due to the need for additional procedures and antibacterial therapy, this can cause a large number of patients and a large amount of medical expenses. In order to evaluate the antibacterial properties of the zwitterionic layer, a hydrophobic polymer (poly(dimethylsiloxane) (PDMS)), a hydrophilic polymer (original hydrophilic PU) and a zwitterionic interpenetrating elastomer The various patches on the non-adhesive surface are incubated with green phosphor. E. coli expressing fluorescent protein (GFP). After 24 hours of incubation, the density of Escherichia coli adhering on each surface was checked using a fluorescence microscope and measured in ImageJ (Figure 3d). In contrast to patches with hydrophobic (≈1370 counts mm-2) and hydrophilic non-adhesive layers (≈1360 counts mm-2), patches with a zwitterionic layer showed significantly lower E. coli adhesion levels (≈0.9 counts mm-2) (Figure 3e).
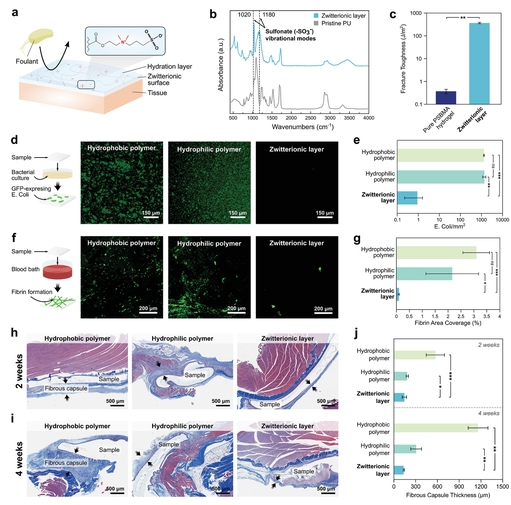
Figure 3 In vitro and in vivo antifouling performance of multilayer patch.
In order to explore the translation potential of multilayer patches in minimally invasive surgery, the author demonstrated two different deployment strategies (balloon catheters and surgical staplers) for applying patches using existing minimally invasive surgical instruments (Figure 4). Due to its paper-thin form and the material properties of its components, the multilayer patch can be customized to adopt a variety of form factors. At room temperature, the dry bioadhesive layer of the patch is in a glass state. As a result, the folded patch can maintain the folded hinge due to plastic deformation, making the patch suitable for origami-based designs (Figure 4a).
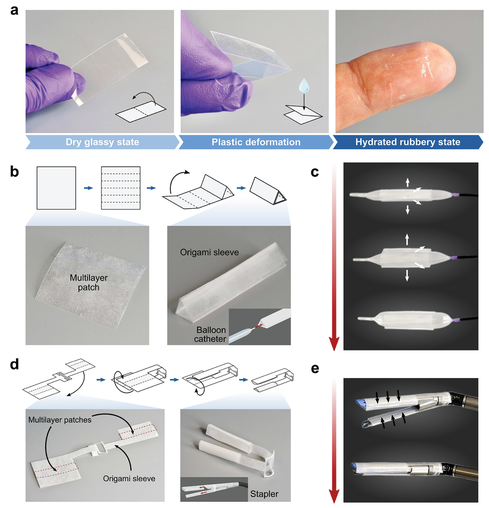
Figure 4 The design and assembly of multilayer patches for various surgical end effectors. a) The photo shows a multilayer patch in a plastically deformable dry glass state. b) Design and manufacture of origami-based triangular sleeves for integrating multi-layer patches and balloon catheters. c) A photograph showing the deployment mechanism using an esophageal balloon catheter. d) The design and manufacture of origami-based double sleeve adapters are used to integrate multi-layer patches with hinged linear staplers. e) A photo showing the unfolding mechanism using an articulated linear stapler.
This minimally invasive application achieved by multi-layer patches is the intraluminal sealing of tubular organs and structures (such as trachea, esophagus and blood vessels) through a balloon catheter. For balloon-based catheter-based delivery and applications, the patch can be folded into a sleeve surrounding an uninflated balloon with the hydrophobic fluid layer facing outward (Figure 4b). When the balloon is inflated, the sleeve will loosen and expand to meet the wall of the hollow organ or blood vessel (Figure 4c). As the inflation pressure of the balloon increases, the radial pressure exerted by the balloon presses the patch against the tissue wall, triggering the deprotection and adhesion of the bioadhesive layer (Figure 5a).
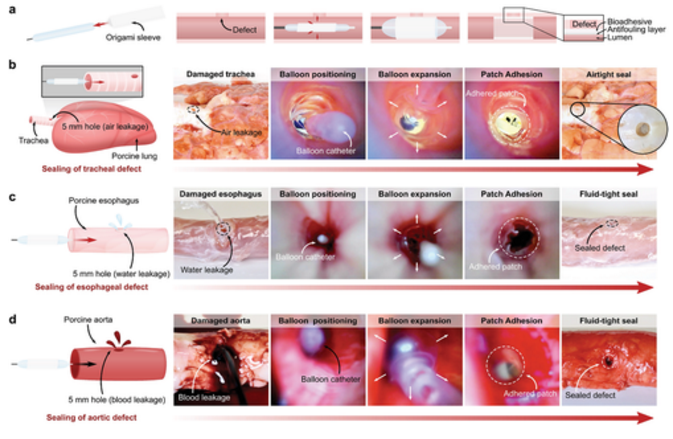
Figure 5 In vitro demonstration of minimally invasive delivery of balloon catheter and multilayer bioadhesive patch. a) Schematic diagram of origami patch integration and intraluminal delivery process using balloon catheter. b) Macro and endoscopic pictures of pig tracheal defect (5 mm hole) airtightly sealed by Foley catheter delivery and application of multilayer patch. c) Macroscopic and endoscopic photos. These photos are patches delivered and applied through the esophageal catheter to liquid-tightly seal the porcine esophageal defect (5 mm hole). d) Macro and endoscopic pictures of porcine aortic defect (5 mm hole) liquid-tightly sealed by Foley catheter delivery and application of patches.
In addition to the intraluminal sealing method using a balloon catheter, the authors also demonstrated that a multilayer patch can be integrated with an arthroscopic stapler (Ethicon) to provide a linear seal (Figure 6). This tissue repair strategy can be used in a wider range of surgical applications, such as anastomosis and resection. In order to realize the minimally invasive delivery based on the stapler, the multi-layer patch is cut into strips of various sizes, and then put into an origami origami sleeve, which is designed to wrap the anvil and the staple bin unit of the stapler (Figure 6a). Once the stapler jaws are positioned near the tissue injury site, the driver of the stapler presses the multilayer patch against the tissue surface, thereby triggering the adhesion and sealing of the defect (Figure 6a). Using this method, a multi-layer patch can quickly and leak-tightly seal a 5 mm round transmural defect in an isolated porcine intestine segment (Figure 6a, b)
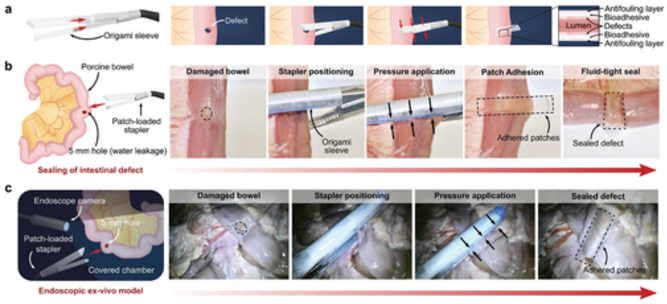
Figure 6 Minimally invasive delivery and application of multilayer bioadhesive patch using surgical stapler for in vitro demonstration. a) A schematic diagram of the patch integration and delivery process using an articulated linear stapler. b) The linear seal of the macroscopic porcine intestinal defect (5 mm hole), which is delivered and administered by a hinged linear stapler. c) Endoscopy is performed in a dark, covered room to seal the porcine intestine defect (5 mm hole) to mimic a minimally invasive surgical procedure.
Link to the paper: doi.org/10.1002/adma.202007667
18915694570
Previous: Acc. Chem. Res. Review