ACS nano: Smart Response Artificial Nerve Hydrogel
The development of modern flexible electronic technology makes bio-electronic materials become artificial tissues in the body. Since hydrogels are more similar to nerve tissue, functional hydrogels have become a promising candidate for bioelectronics. At the same time, the interface between functional hydrogels and living tissues is at the forefront of bioelectronics. Peripheral nerve injury often leads to paralysis, chronic pain, neurological disorders and even disability, because it affects the transmission of bioelectric signals between the brain and other parts of the body.
Recently, the team of Professor Shen Qundong from Nanjing University in Jiangsu has developed a conductive polymer hydrogel (CPH) with light stimulation response and stretchability to explore artificial nerves. Near-infrared light irradiation can increase the conductivity of CPH and promote the conduction of bioelectric signals. When CPH is mechanically extended, it still has high conductivity durability, and therefore, can accommodate accidental strains of nerve tissue during exercise. Related work was published in the journal ACS Nano with the title "Conductive Hydrogel for a Photothermal-Responsive Stretchable Artificial Nerve and Coalescing with a Damaged Peripheral Nerve".
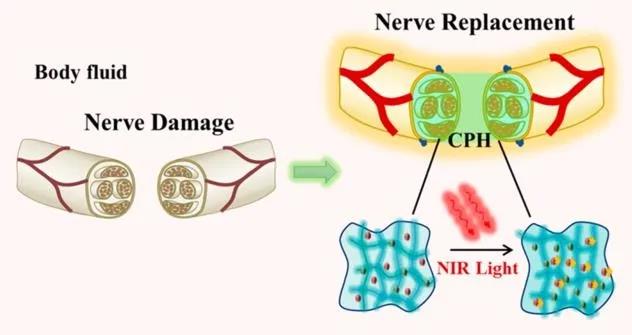
Figure 1 Highly flexible and near-infrared response conductive polymer hydrogel to replace damaged peripheral nerves
Through the copolymerization of polyaniline (PANI) and polyacrylamide (PAM), a tough conductive hydrogel with excellent electrical conductivity, mechanical properties similar to nerve tissue, and good biocompatibility was prepared. The amide bond of PAM can interact with aniline molecules, which can adsorb and induce the polymerization of aniline in situ to form a transparent PAM/PANI conductive gel. After freeze-drying, the cross-sectional morphology of CPHs was observed by scanning electron microscope (SEM). Similar to typical hydrogels, CPHs exhibit a three-dimensional microporous foam network. Conducive to cell penetration and tissue formation within the three-dimensional hydrogel structure (Figure 2B, C). At the same time, due to the doping of PANI, the prepared CPHs showed good electrical properties, even if they were extended to three times the original length (Figure 2E, F).
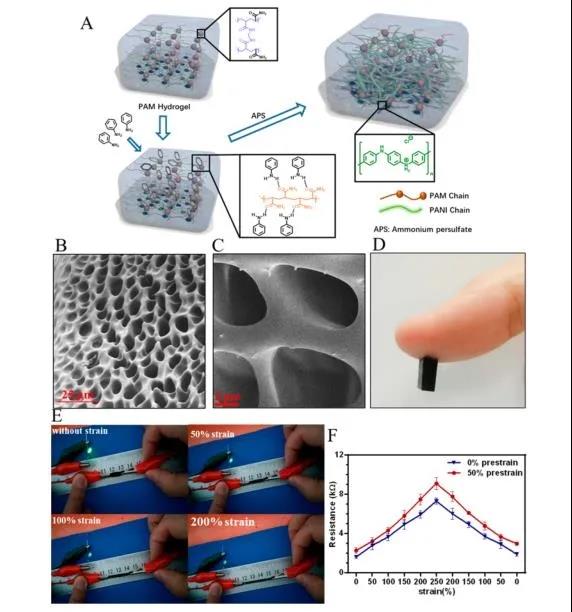
Figure 2 Preparation and characterization of conductive polymer hydrogel
As shown in Figure 3, the resistivity of CPH (0.2-0.3Ω·m) is much better than PAM, which is due to the doping of polyaniline. Electrochemical impedance spectroscopy (EIS) detects the electrical activity of CPH in PBS. As shown in Figure 3B, the Nyquist diagrams of PAM and CPH are quasi-semi-circular in the high-frequency region, indicating that both PAM and CPH have the ability of charge transfer. In addition, the feasibility of CPH replacing damaged nerves in vivo and the conduction of bioelectric signals in nerves are also simulated. As shown in Figure 3E, when the nerve is completely damaged, the bioelectric signal cannot be transmitted. After the damaged nerve is connected to the CPH, the bioelectric signal transmission function can be restored.
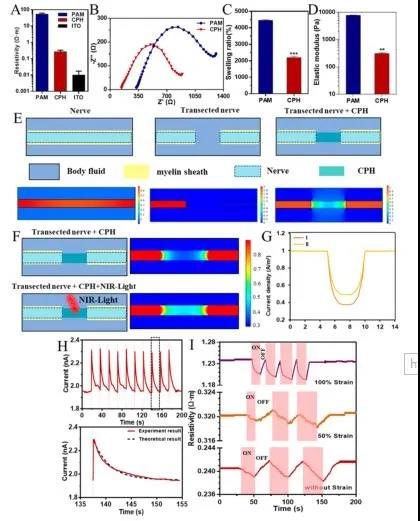
Figure 3 Physical and electrical properties of CPH
Subsequently, the author selected the isolated sciatic nerve and gastrocnemius muscle to verify whether CPH has good electrical conductivity and can be used as an in vitro implantable nerve substitute. The CPH replacement nerve group showed the same increasing trend as the sciatic nerve group with the increase of stimulation voltage (Figure 4D). In addition, the nerve conduction time (CT) of the sciatic nerve after CPH replacement is similar to that of the intact sciatic nerve, suggesting that CPH can replace the damaged nerve functionally (Figure 4E). This phenomenon further proves that CPH can replace damaged nerves and restore secondary sensory function.
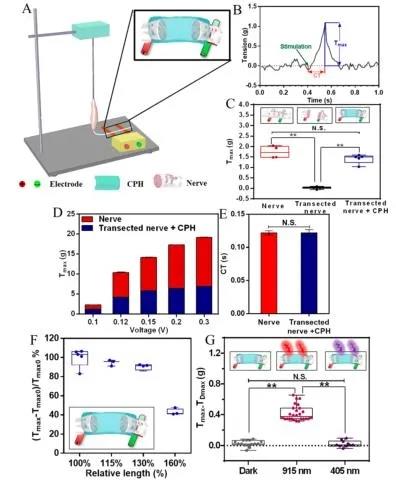
Figure 4 Replacement of toad sciatic nerve with CPH in vitro
In order to simulate peripheral nerve injury, the sciatic nerve was exposed through a split incision of the lateral dorsal gluteus muscle and a 10 mm sciatic nerve was removed. Then, using the linear CPH prepared for the implant, the injured nerve is sutured to the nerve. As shown in Figure 5B and C, after single-phase pulse stimulation, the peak symmetry of CMAPs in the transverse nerve group is significantly different from that of normal nerves. The CPH replacement group has similar peak symmetry with normal nerves, indicating that CPH can replace damaged nerves to achieve bioelectric signal transduction.
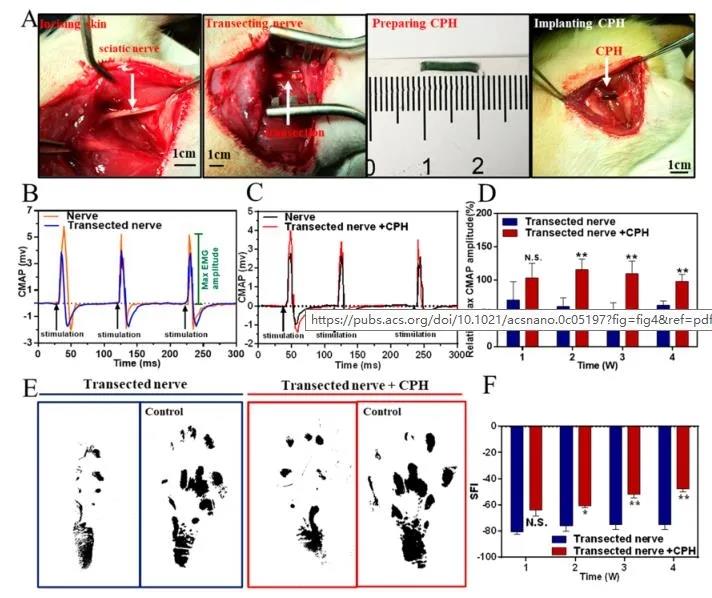
Figure 5 Used for surgical implantation, surgery and replacement of the sciatic nerve in a rodent model
In summary, the author prepared a tough conductive CPH with excellent conductivity, mechanical properties similar to nerve tissue, and good biocompatibility through the copolymerization of PANI and PAM. These excellent properties of CPH successfully replaced the damaged snake-footed toad sciatic nerve in vitro. In addition, our in vivo data show that implantation of CPH as a highly conductive bridge can replace the loss of sciatic nerve, indicating that CPH can be used as a potential biomaterial to replace damaged peripheral nerves in tissue engineering.
Information source: EngineeringForLife
This information is from the Internet for academic exchanges. If there is any infringement, please contact us and delete it immediately
Recently, the team of Professor Shen Qundong from Nanjing University in Jiangsu has developed a conductive polymer hydrogel (CPH) with light stimulation response and stretchability to explore artificial nerves. Near-infrared light irradiation can increase the conductivity of CPH and promote the conduction of bioelectric signals. When CPH is mechanically extended, it still has high conductivity durability, and therefore, can accommodate accidental strains of nerve tissue during exercise. Related work was published in the journal ACS Nano with the title "Conductive Hydrogel for a Photothermal-Responsive Stretchable Artificial Nerve and Coalescing with a Damaged Peripheral Nerve".
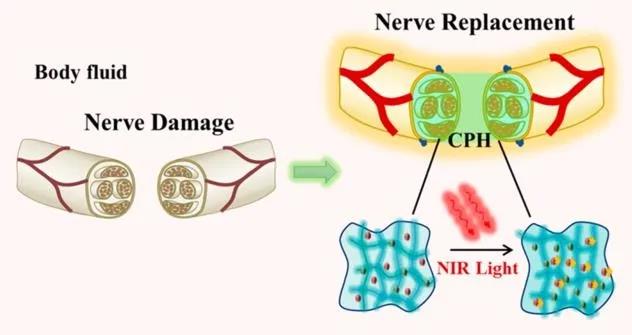
Figure 1 Highly flexible and near-infrared response conductive polymer hydrogel to replace damaged peripheral nerves
Through the copolymerization of polyaniline (PANI) and polyacrylamide (PAM), a tough conductive hydrogel with excellent electrical conductivity, mechanical properties similar to nerve tissue, and good biocompatibility was prepared. The amide bond of PAM can interact with aniline molecules, which can adsorb and induce the polymerization of aniline in situ to form a transparent PAM/PANI conductive gel. After freeze-drying, the cross-sectional morphology of CPHs was observed by scanning electron microscope (SEM). Similar to typical hydrogels, CPHs exhibit a three-dimensional microporous foam network. Conducive to cell penetration and tissue formation within the three-dimensional hydrogel structure (Figure 2B, C). At the same time, due to the doping of PANI, the prepared CPHs showed good electrical properties, even if they were extended to three times the original length (Figure 2E, F).
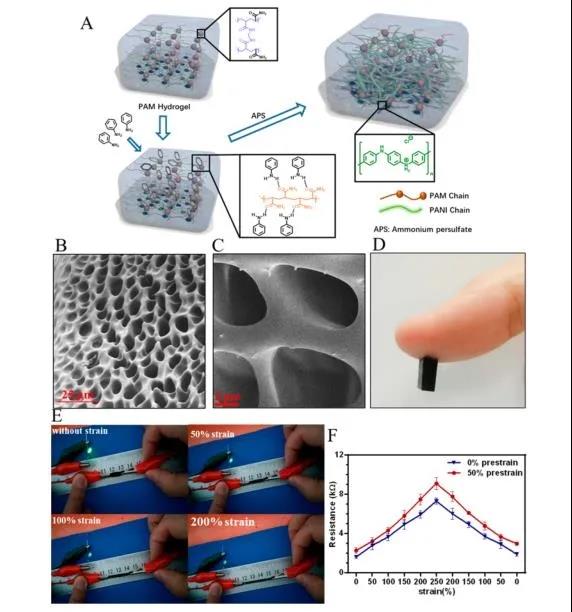
Figure 2 Preparation and characterization of conductive polymer hydrogel
As shown in Figure 3, the resistivity of CPH (0.2-0.3Ω·m) is much better than PAM, which is due to the doping of polyaniline. Electrochemical impedance spectroscopy (EIS) detects the electrical activity of CPH in PBS. As shown in Figure 3B, the Nyquist diagrams of PAM and CPH are quasi-semi-circular in the high-frequency region, indicating that both PAM and CPH have the ability of charge transfer. In addition, the feasibility of CPH replacing damaged nerves in vivo and the conduction of bioelectric signals in nerves are also simulated. As shown in Figure 3E, when the nerve is completely damaged, the bioelectric signal cannot be transmitted. After the damaged nerve is connected to the CPH, the bioelectric signal transmission function can be restored.
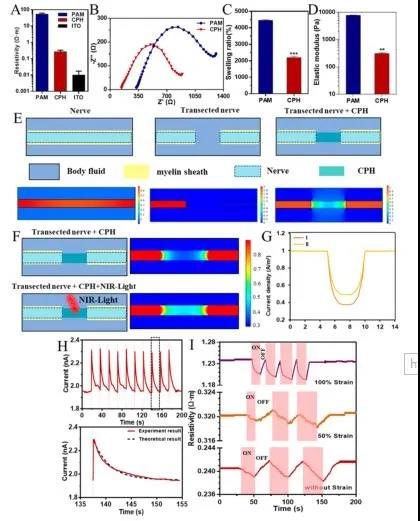
Figure 3 Physical and electrical properties of CPH
Subsequently, the author selected the isolated sciatic nerve and gastrocnemius muscle to verify whether CPH has good electrical conductivity and can be used as an in vitro implantable nerve substitute. The CPH replacement nerve group showed the same increasing trend as the sciatic nerve group with the increase of stimulation voltage (Figure 4D). In addition, the nerve conduction time (CT) of the sciatic nerve after CPH replacement is similar to that of the intact sciatic nerve, suggesting that CPH can replace the damaged nerve functionally (Figure 4E). This phenomenon further proves that CPH can replace damaged nerves and restore secondary sensory function.
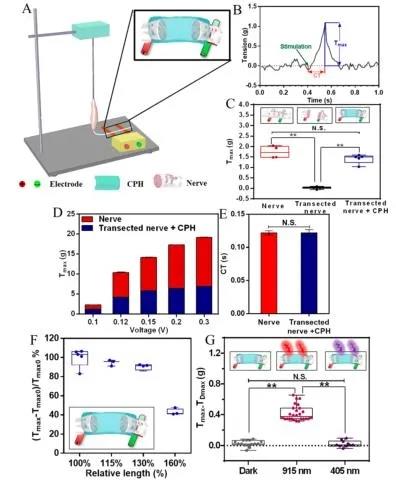
Figure 4 Replacement of toad sciatic nerve with CPH in vitro
In order to simulate peripheral nerve injury, the sciatic nerve was exposed through a split incision of the lateral dorsal gluteus muscle and a 10 mm sciatic nerve was removed. Then, using the linear CPH prepared for the implant, the injured nerve is sutured to the nerve. As shown in Figure 5B and C, after single-phase pulse stimulation, the peak symmetry of CMAPs in the transverse nerve group is significantly different from that of normal nerves. The CPH replacement group has similar peak symmetry with normal nerves, indicating that CPH can replace damaged nerves to achieve bioelectric signal transduction.
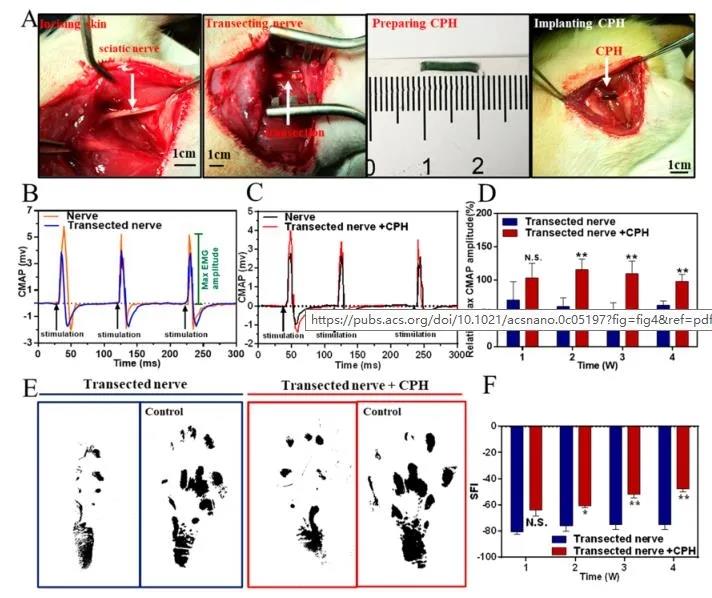
Figure 5 Used for surgical implantation, surgery and replacement of the sciatic nerve in a rodent model
In summary, the author prepared a tough conductive CPH with excellent conductivity, mechanical properties similar to nerve tissue, and good biocompatibility through the copolymerization of PANI and PAM. These excellent properties of CPH successfully replaced the damaged snake-footed toad sciatic nerve in vitro. In addition, our in vivo data show that implantation of CPH as a highly conductive bridge can replace the loss of sciatic nerve, indicating that CPH can be used as a potential biomaterial to replace damaged peripheral nerves in tissue engineering.
Information source: EngineeringForLife
This information is from the Internet for academic exchanges. If there is any infringement, please contact us and delete it immediately
18915694570
Previous: Nature Medicine: Zwitt